Induction heating is a powerful and versatile technology that has revolutionized a wide range of industrial processes. This technology offers numerous advantages over standard heating methods. It’s a clean, contactless, and efficient way to heat conductive materials. Furthermore, induction heating is especially useful for performing very precise or repeatable heating operations. In this blog post, we will look at how induction heating works and how it is used in various industries.
Induction heating working principle
In an induction heating system, an alternating current (AC) is passed through a coil. As a result, an alternating magnetic field is generated by the coil. When a conductive object is placed in this field, it may get heated due to hysteresis and/or eddy current losses.
Hysteresis losses
To understand hysteresis losses, we must first recall what a magnetic hysteresis loop is. A magnetic hysteresis loop shows the relationship between the magnetizing force (H) and the induced magnetic flux density (B). Figure 1 shows an example of a hysteresis loop.
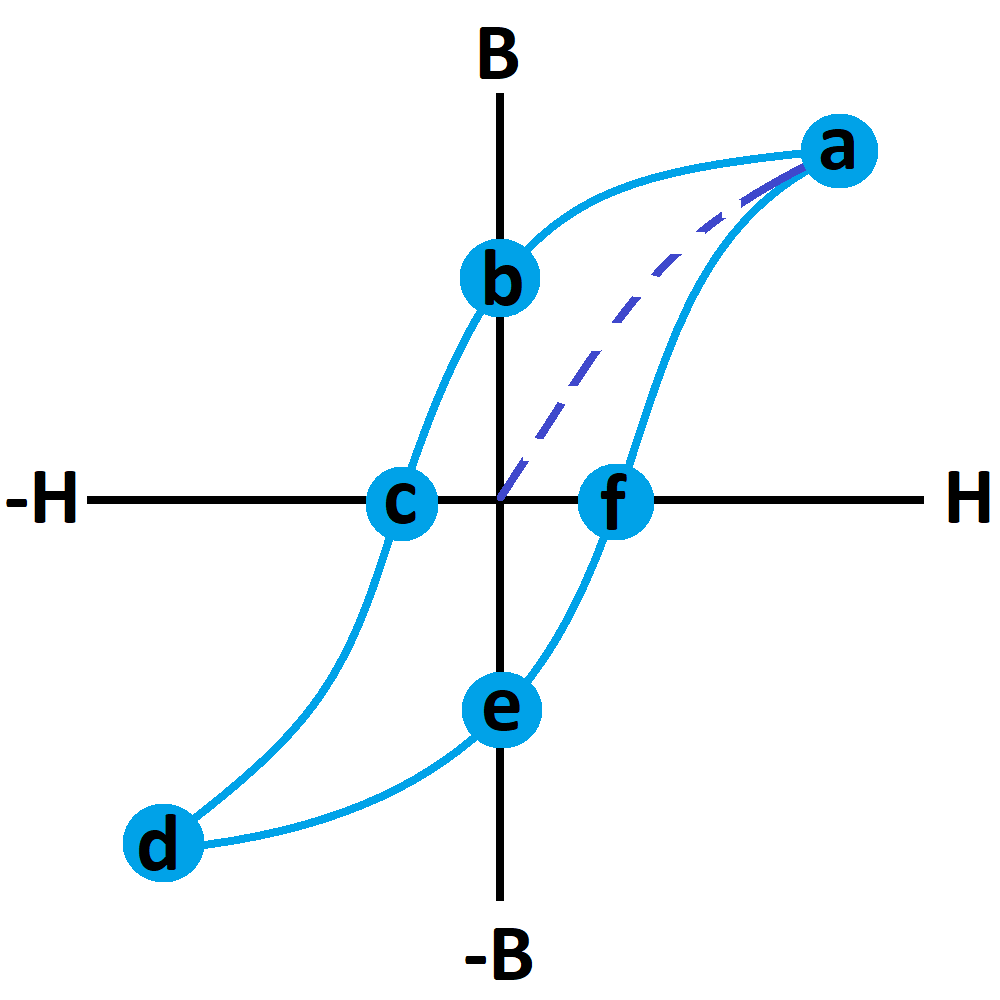
A ferromagnetic material that is not magnetized will follow the dashed line as the magnetizing force increases (Figure 1). This line shows that the greater the magnetizing force is applied, the bigger the flux density (B) is produced in a material. At point “a,” the material will reach magnetic saturation. At this point, a further increase in magnetizing force will result in a relatively minor increase in magnetic flux.
If a ferromagnetic material is magnetized in one direction and the imposed magnetizing field is withdrawn, it does not relax back to zero magnetization. So when H is reduced to zero, the curve moves from point “a” to point “b.” Even when the H is zero, some magnetic flux (B) persists in the material. In order to demagnetize the material, the magnetizing force must be reversed.
When the magnetizing force is applied in the opposite direction (-H), the curve moves to point “c,” where the magnetic flux (B) is zero. This is known as the point of coercivity. It demonstrates how much reversed magnetizing power is required to zero out the net flux within the material.
As the reversed magnetizing force (-H) is increased, the curve will move to point “d” where the material will become magnetically saturated.
When the reversed magnetizing force is reduced to zero, the curve reaches point “e.” It will have the same amount of residual magnetism as in point “b.” Increasing H back in the positive direction will return B to zero (point “f”). Because some force is necessary to eliminate the remaining magnetism, the curve did not return to the graph’s origin. To reach saturation point “a,” the curve will now take a different path than before.
As explained previously, a coercive force must be applied to overcome residual magnetism. The energy necessary to close the hysteresis loop will be dissipated as heat in the magnetic material. This is known as hysteresis loss. The amount of loss depends on the material’s value for coercive force. Only magnetic materials experience hysteresis losses.
Another thing that should be mentioned is the Curie temperature. It is the temperature at which some magnetic materials suffer a dramatic shift in their magnetic characteristics. The Curie temperature of iron, for example, is 770 °C (1390 °F). When iron is heated over this temperature, it loses its magnetic properties. Hence, at that point, we can’t rely on hysteresis for heating. Moreover, when steel is heated below the Curie threshold, the effect of hysteresis loss is generally so minor that it can be ignored.
Eddy current losses
Eddy currents are electrical current loops caused by a changing magnetic field within a conducting material. These currents run through conductors in closed loops in planes perpendicular to the magnetic field (Figure 2). The size of the current in a particular loop is proportional to the strength of the magnetic field, the area of the loop, and the rate of change of flux, and inversely related to the resistivity of the material.
The current flowing through the resistance of the conductor dissipates energy as heat in the material. Induction heating devices use this heating effect. The resistance felt by the eddy currents in a conductor causes Joule heating. The amount of heat generated is proportional to the square of the current. I should note that eddy current causes heating even if the materials do not have any magnetic properties. In induction heating, eddy current losses are far more significant than hysteresis losses.
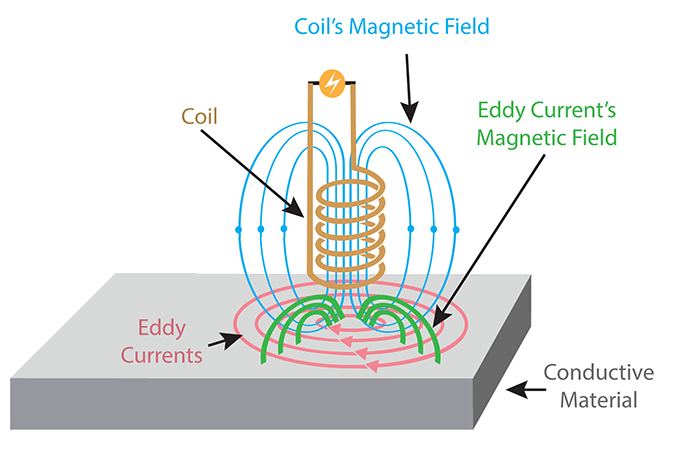
Eddy currents are also affected by the frequency of the magnetic field. At high frequencies, the current flows close to the conductor surface. This is due to the skin effect. This effect is used to control the penetration depth of the induction heating process. As a result, the entire object or only its surface can be heated. It should be made clear that the skin effect is also observed inside the induction coil conductor.
How does my induction hob work?
Under the induction hob’s surface there are induction coils made from copper wire (Figure 3). Hob’s control circuit sends high-frequency (20–50 kHz) alternating current through the coil. The current in the coil generates an electromagnetic field. This field induces eddy currents in any conductive material that is placed on the hob’s surface. The induction hob’s coil has numerous turns, whereas the bottom of the pot practically makes a single turn. This forms a transformer that steps down the voltage while increasing the current. The eddy current flowing through the resistance of the electrically conductive pot dissipates energy as heat. This is known as the Joule heating effect. When heat is generated in cookware, it is transferred to the food inside.
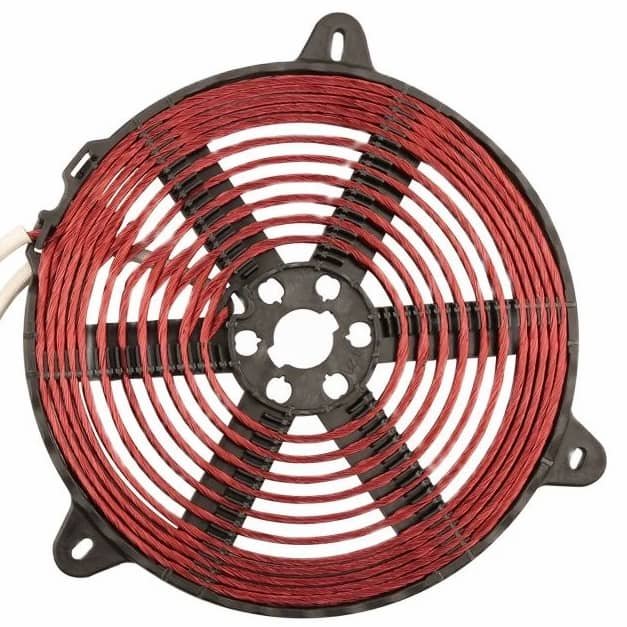
Summary
Induction heating is a versatile technology that relies on the principle of electromagnetic induction to generate heat in a conductive material. The main heat source in induction heating is eddy current losses. These currents cause the Joule heating effect in conductive materials. Induction heating has many advantages over traditional heating methods. Its ability to control the amount and depth of heating and target specific areas of a conductive material make it a valuable tool for many industrial applications.